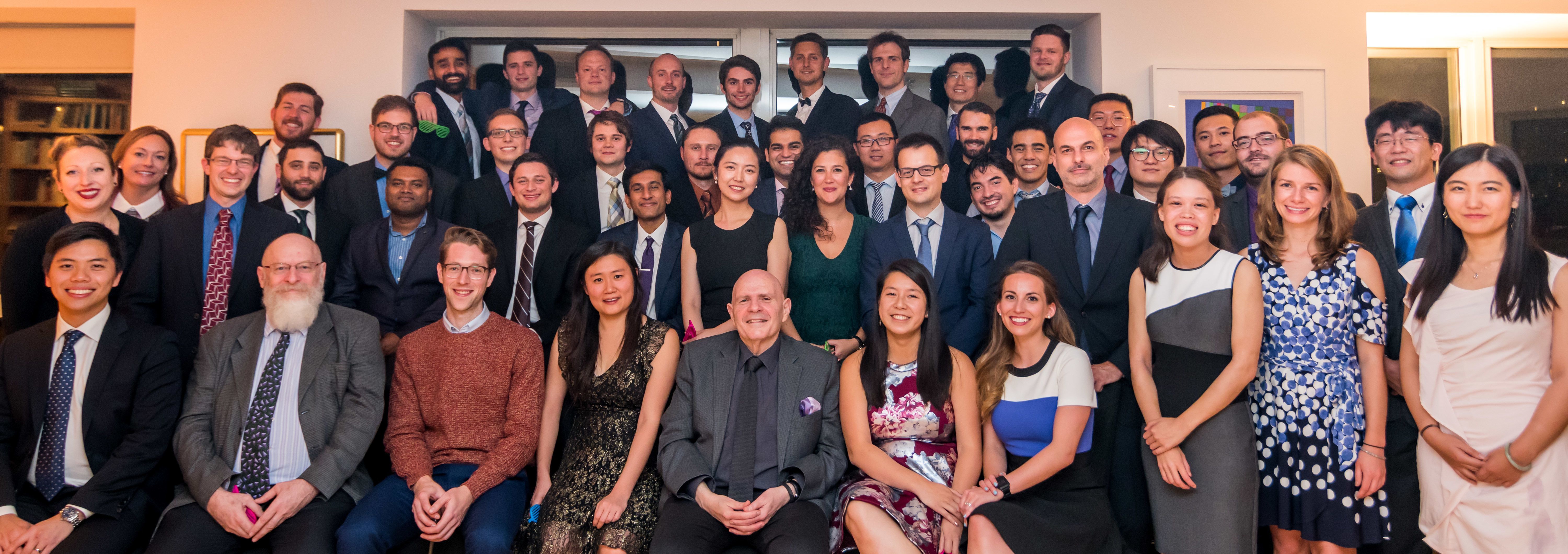
(pictured above: Members of the Stupp Laboratory, at Northwestern University)
My default is to roll my eyes when a set of relatives and friends all start sending me the same news blurb for another spinal cord injury study. From reading the press release, you can never tell if this new finding is worth getting excited about. It’s rare that a piece of research lives up to the hype that a university press machine is drumming up. But I must admit, the work published by the Samuel Stupp lab (Alvarez et al., 2021) in Science this November, does appear promising.
In this study from a materials engineering group, a bioactive scaffold was injected into lesion sites of mice that received a severe, contusive injury – an injury model that best mimics human lesions – and led to significant recovery, including walking. This is the second paper from the group using their dynamic bioscaffolds to treat spinal cord injury. The first was published in 2008 in a middle-tier journal without much fanfare. So, what changed in 13 years that earned a publication in Science and all those news blurbs?
First, they changed the structure of their polymers and found that the molecules that formed the most dynamic assemblies had the most potent effects on cells in culture dishes. The exact mechanism of why this is the case remains to be established. But the authors went on to test their new, more dynamic molecules in injured mice.
In addition to improving the structure to be more potent, the authors also expanded the repertoire of the bioactive sites on the polymers. In their first papers, the authors characterized polymers that had a protein piece from the structural protein laminin. Neurons love to grow axons along laminin. When scientists grow neurons in dishes, they need to coat the plastic in laminin so the neurons attach and extend their processes. Laminin is an important part of the extracellular matrix – the dense network of proteins between cells that provides support and structure. By including a piece of the laminin protein in their bioscaffold, the authors are trying to make it look like a growth-promoting extracellular matrix to neurons. A new addition to the polymers for this paper is a piece of fibroblast growth factor 2 (FGF2). FGF2 is a signal that promotes cell survival and cell division.
The authors also changed the injury model for this paper. In 2008, they used a crush model. To do a crush you squeeze the spinal cord from both sides with a clip or forceps. This model has been used extensively because it’s so easy to do and very reproducible from mouse to mouse. It forms a relatively small lesion, though. As a result, the distance that regenerating neurons need to grow to span the gap is very short. It also has a problem with “sparing”: sometimes neurons survive the crush and the injury is not complete. The lesion also often features “astrocyte bridges” – tracts of cells that regenerating neurons can use to regrow. Seeing regeneration in a crush model is not very convincing, overall. The bar for regeneration is low for this model. And all those factors that help regeneration in the crush model are not present in the lesions of human patients.
For the present study, the authors used a severe contusion model. This involves dropping a weight onto the spinal cord. The contusion model is widely accepted as the best clinical model. After a severe contusion, there is very little spontaneous recovery and the gap that the regenerating neurons need to cross is significantly larger. In mice, this is roughly 2 nanometers. A human spinal cord lesion is around 2-3 centimeters in length. This is a distance 10 million times longer than the mouse lesion. So, we have a way to go for human efficacy…
The authors injected this new version of their dynamic bioactive scaffold into the injury site 24 hours after contusion. This is a clinically relevant time frame and one characterized by massive cell death and inflammation. They saw four key areas of improvement, which were the most dramatic in the animals that got the most dynamic combination of the laminin and the FGF2 polymers.
First, the bioactive polymers resulted in better neuronal survival at the site of injury. This result is very dramatic. It seems that the polymers have a neuroprotective effect. Without polymer injection, basically zero neurons survived at the site of contusion. With polymer injection, about 300 neurons were present in a slice. While a normal slice has about 700 neurons. This represents a big improvement at the site of injury.
Second, and this is an observation that probably helps to explain the first, new blood vessels grew at the site of injury with bioactive polymer injection. These blood vessels helped to support the survival of neurons in the lesion.
Third, the axons of cut corticospinal neurons regenerated into the site of injury and a small number of axons made it all the way across the lesion and into the spared tissue on the other side. This is significant because the corticospinal tract (CST) is absolutely vital for voluntary movement in humans and is also one of the most resistant to regenerative stimuli. Seeing such robust growth of the CST is striking and very encouraging for this treatment.
Fourth, most significantly, the severely contused mice showed drastic recovery with injection of the bioactive polymers. Using a standardized metric to measure recovery after SCI, the authors saw their polymer-treated animals score 6 out of 9 (uninjured) on the BMS scale. Mice that did not receive the bioactive polymer injection scored only a 2. This represents a difference between hindlimb movement with no weight-bearing or stepping to walking with correct foot placement but showing issues with coordination and trunk stability. This is another significant result. To see so much recovery after a severe contusion is incredibly rare.
What could be the mechanism underlying this recovery? Though this has not been proven, I think the main reason for the function recovery seen is the neuroprotective function of the polymers. I don’t think the regeneration by the CST (regeneration that is undoubtedly also occurring in other tracts of the spinal cord, though not quantified in the study) is contributing because all of the recovery happens very quickly. By week 3 post-injury, the recovery has already plateaued in all treatment groups. This is not on the time frame of new axons growing and new connections being made. In their previous study, they showed that hardly any neurons penetrate the lesion even two weeks after injury. The CST growth that they show is from a time point 12 weeks after injury. Plus, so few CST neurons make it all the way across the injury site, it is unlikely that these new connections would make a big functional difference.
So, what does this mean for human patients? Like I said, I am encouraged and excited by this work. But I think it’s clear that only newly-injured individuals would likely benefit from an injection of these bioactive polymers. Right after injury, there is a narrow window during which neuronal cell death can be prevented. In chronic cases, neuroprotection would not make an impact on function because all the neurons have already died.
In addition, it seems unlikely that an intervention that promotes growth of the CST on the nanometer scale would help human axons cross a lesion 10 million times longer. One way to get around this problem would be to combine bioactive scaffolds with stem cell transplants. If stem cells filled the lesion and differentiated into neurons that made connections below the injury site, the CST above the injury site would not have to extend axons all the way across the lesion. Instead, a neural relay can be formed. Such a combination therapy could be beneficial in chronic injuries. Suggesting such a combination therapy is also reasonable since in their first paper characterizing their bioactive scaffolds, the authors showed that their matrix promotes the maturation of stem cells into neurons and the extension of neuronal processes in culture.
Lastly, I want to stress that the bioactive scaffold injection will likely do more than just promote motor recovery, though that was the focus of the paper. In their previous study, the authors showed that sensory axons grow up into the treated lesion from the bottom. And the treatment promoted survival of more than just motor neurons. So, overall, though the study focused on movement, sensory and autonomic function could also potentially be improved by this intervention. I am looking forward to the follow-up work from this group. And I expect clinical trials of this bioactive polymer are not far away.
PS - Have an SCI topic, treatment or study you'd like me to address in a future article? Respond to this article with your suggestions.
Alina Garbuzov is a stem cell biologist and geneticist with a PhD from Stanford University School of Medicine; she suffered an SCI while rock climbing in 2015. She currently holds a post-doctoral position in Mark Tuszynski’s lab at the University of California, San Diego. Dr. Garbuzov presented on neural stem cell grafts at U2FP's Annual Symposium in 2019 (watch her presentation here). She is an occasional science contributor for U2FP, using this space to explore scientific developments in the spinal cord injury field in order to drive smart decision-making both for personal health and for setting research goals.